Transport across Cell Membranes
transport across Cell Membranes
The substances move through the cell membrane by two major processes: passive and active. The passive transport requires no energy; the active transport, on the other hand, does consume energy.
Passive Transport Processes
In passive transport processes, the substances move across the cell membrane without any energy expenditure by the cell. It includes diffusion and osmosis.
A. Diffusion
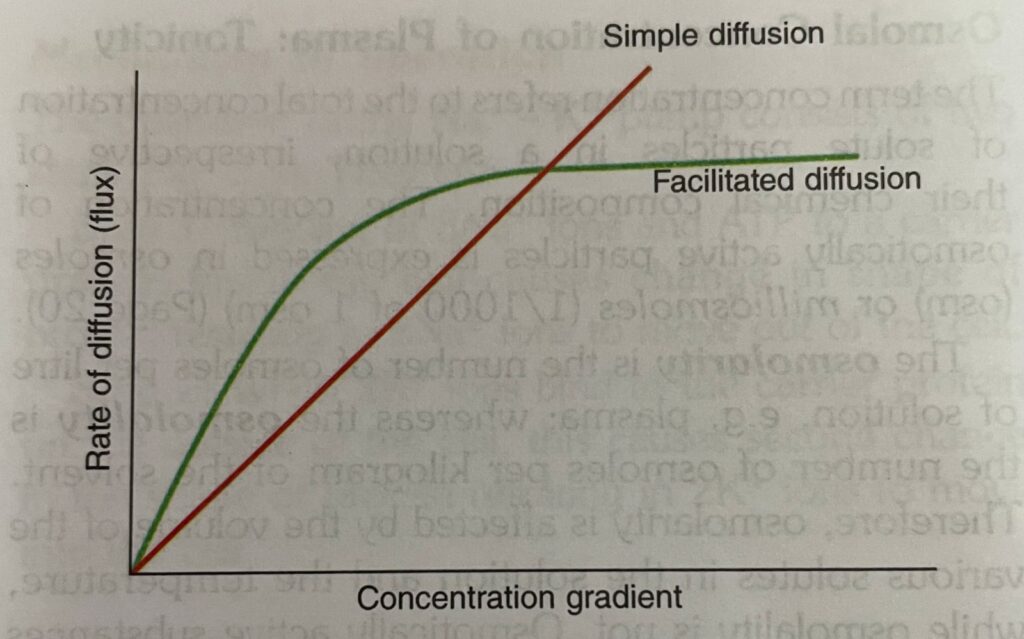
Diffusion is a passive process i.e. no external source of energy is required) by which molecules move from areas of high concentration to areas of low concentration (down their ‘chemical gradient’).
The cations (positively charged molecules) move to negatively charged areas whereas anions move to positively charged areas (down their electrical gradient’). It is of two types: simple diffusion and facilitated diffusion.
1.Simple Diffusion
Characteristic features
(i) Simple diffusion occurs because the heat content of the solution keeps the solvent and the solute particles of the solution in constant motion.
(ii) The net movement ceases when the concentration of the molecules is equal everywhere within the solution (diffusional equilibrium).
(iii) Factors affecting diffusion: The ‘rate’ at which a material diffuses through a membrane (flux) is given by Fick’s law of diffusion, i.e.
Net rate of diffusion = (-) [D X A / T] x (Cin-Cout)
D- diffusion co- efficient , A- area of membrane, T- Thickness of Membrane, Cin and Cout = Concentration of the material inside and outside of the membrane. The negative sign indicates that the material is moving down its concentration gradient.
(a) Distance: The greater the distance, the longer is the time required
(b) Size of the gradient: The larger the concentration gradient, faster the diffusion proceeds.
(c) Temperature: The higher the temperature, faster is the diffusion rate. At normal body temperature of 37 °C, diffusion is optimal (maximum).
(d) Molecular size: The permeability of cell membrane to a substance falls rapidly with increase in molecular weight in the range between 10,000 and 60,000. This is why glucose diffuses faster than large proteins.
(e) Lipid solubility: Lipid soluble molecules (02 CO2, N2) diffuse rapidly with ease through the lipid layer of the membrane. Water soluble molecules (ions, glucose, urea) can cross the cell membrane slowly.
Important Note
‘Ions’ also utilize ionic channels to cross the cell membrane. Some channels are continuously open, whereas others are ‘gated.
Gated channels have gates that open or close either
(i) by alteration in membrane potential (voltage gated), e.g. Nat and Ca?+ channels; or
(ii) when they bind a ligand, i.e. either an ion or a specific molecule (ligand gated). The ligand is either external (e.g. neurotransmitter or a hormone) or internal (e.g. intracellular Ca?+ cAMP, or G protein produced in the cells). Some channels are also opened by mechanical stretch (mechanosensitive channel).
Important Note
The extracellular ligands are called first messengers and the intracellular mediators are called second messengers.
The second messengers generally activate protein kinases.
2. Facilitated Diffusion

Characteristic features
- (i) Facilitated diffusion is a carrier-mediated process that enables molecules which are too large to flow through membrane channels by simple diffusion (Fig. 2.1). For example,
- (a) glucose transport by the glucose transporter (GLUT) across intestinal epithelium.
- (b) the transport of glucose into RBCs, muscles and adipose tissues in the presence of insulin.
- (ii) The carrier protein undergoes repetitive spontaneous configurational changes during which the binding site for the substance is alternately exposed to the ICF and ECF.
- (iii) Its rate of diffusion increases with increase in concentration gradient to reach a plateau when all the binding sites on the carrier proteins are filled. This is called saturation
Important Note
Diffusion is a major force that affects the distribution of water and solutes in different body compartments.
Some substances (weak acids and weak bases) which are quite soluble in cell membranes in undissociated form, and cross the membrane with difficulty in the ionic form. However, if an undissociated substance diffuses from one side of the membrane to the other end and then dissociates, there occurs net movement of the undissociated substance from one side of the membrane to another, called non-ionic diffusion.
This phenomenon is seen in the gastrointestinal tract (GIT) and kidneys.
B. Osmosis

Definition: Osmosis is the passive flow of the solvent, e.g. water across a selectively permeable membrane (i.e. membrane permeable to solvent but not to the solute) into a region where there is higher concentration of a solute to which the membrane is impermeable.
Osmotic Pressure
The tendency for movement of solvent molecules to pass across a membrane from a low concentration of solute to a region of greater solute concentration can be prevented by applying pressure to the more concentrated solution. The amount of pressure exactly required to prevent solvent migration (i.e. osmosis) is called osmotic pressure of the solution .
Osmotic pressure of a solution is related to the following:
(1) Number of particles (molecules or ions) dissolved in the solution rather than their size, type, molecular weight or chemical composition
(2) Temperature and volume in the same way as the pressure (P) of a gas, i.e.
P = nRT/V
where, n = number of particles, R = gas constant, T = absolute temperature and V =volume of solution
Osmolal Concentration of Plasma: Tonicity
The term concentration refers to the total concentration of solute particles in a solution, irrespective of their chemical composition. The concentration of osmotically active particles is expressed in osmoles (osm) or milliosmoles (1/1000 of 1 osm) .
The osmolarity is the number of osmoles per liter of solution, e.g. plasma; whereas the osmolality is the number of osmoles per kilogram of the solvent. Therefore, osmolarity is affected by the volume of the various solutes in the solution and the temperature, while osmolality is not. Osmotically active substances in the body dissolve in water. As the density of water is 1, so osmolal concentration is expressed as osm/L of water.
The osmolality of normal human plasma is 290 mosm/L. The osmolality of a solution relative to plasma is called tonicity. Solutions that have the same osmolality as plasma are said to be isotonic (eg. 0.9% sodium chloride solution or 5% glucose solution).Those solutions which have greater osmolality are called hypertonic; and those with lesser osmolality are called hypotonic.
Relative contribution of the various plasma components to the total osmolal concentration of plasma:
- Approximately 270 of the 290 mosm/ L of normal plasma are contributed by Nat and its accompanying anions, mainly CF and HCO; because there is no net movement of these osmotically active particles into the cells and these particles are not metabolised.
- Although the concentration of the plasma proteins is large (average 6.4-8.3 g/dL), they normally contribute less than 2 mosm/L because of their very high molecular weights.
- The major non-electrolytes of plasma, glucose and urea which are in equilibrium with the cells contribute about 5 mosm/L. But this can be quite large in hyperglycaemia or uraemia.
Clinical significance
The total plasma osmolality is important in assessing dehydration, overhydration, and other fluid and electrolyte abnormalities. For example:
- Hyperosmolality can cause coma (hyperosmolar coma by causing water to flow out of the cells, i.e. cellular dehydration.
- The flow of water in or out of the capillaries depends on whether the colloidal osmotic pressure or oncotic pressure (osmotic pressure produced by plasma proteins) is greater or lesser than the hydrostatic pressure of the blood (produced by systemic arterial blood pressure). When water flows into or out of capillaries, it carries dissolved particles with it. This force is called solvent drag.
Its effects are very small in the body.
Important Note
Clinically, a rough estimation of plasma osmolality can be made by using the following formula.
Osmolality (mosm/L) = 2mEq/L [Na+] + 0.055mg/dl [glucose] + 0.36mg/dl [Blood urea]
Active Transport Processes
In active transport processes the substances are transported against their chemical and electrical gradient. This form of transport requires energy and is called active transport. It includes:
(A) Primary active transport processes
(B) Secondary active transport processes
(C) Carrier type processes
(D) Vesicular transport processes
Important Note
As the active transport processes require energy, they are often referred to as pumps.
A. Primary active transport processes
The primary active transport processes directly use the energy obtained from the hydrolysis of adenosine triphosphate (ATP). The primary active transport system consists of:
- Sodium-potassium (Na+ – K+) pump
- Calcium (Ca?+) pump
- Potassium hydrogen (K+ – H+) pump
Sodium-potassium (Na+ – K+) pump or Na + – K+ ATPase
The sodium-potassium pump is the most common pump found in all parts of the body. It uses the membrane-bound ATPase as a carrier molecule, i.e. an enzyme that catalyses the hydrolysis of ATP. One of these ATPase is sodium-potassium activated ATPase (Na+ – K+ ATPase).
Structure
ATPase is composed of six sub-units, three a and three ß. All these sub-units extend through the membrane of most cells. Sodium and potassium transport occurs through a sub-unit. a sub-unit has
(i) ATPase enzymatic activity, i.e. the ability to convert ATP to adenosine diphosphate (ADP), thereby releasing energy.
(ii) binding sites on its intracellular and extracellular faces. The former contains binding sites for three Na+ ions and an AT molecule whereas the latter contains binding sites for two K+ ions
Mechanism of operation

The operation of the Na+ – K+ pump consists of two steps. They are:
Step 1: Binding of 3Na+ ions and ATP to a carrier protein inside the cell causes change in shape of protein resulting in 3Na+ ions to move out of the cell.
Step 2: When 2K+ ions bind to the carrier protein on the outside of the cell, this causes second change in the shape of protein resulting in 2K+ ions to move into the cell.
Functions
- Na+ – K+ pump is responsible to maintain the high K+ and low Nat concentrations in the cell. How?
Na+ – K+ pump catalyzes the hydrolysis of ATP to ADP and uses the energy to force out 3Na+ ions from the cell and take 2K+ ions into the cell for each mole of AT hydrolysed. Thus it is an electrogenic pump with a coupling ratio of
3: 2 and produces net movement of positive charge out of the cell.
- Active transport of Na+ ions and K+ ions is one of the major energy-using processes in the body. It accounts for a large part of the basal metabolism.
- It is useful in regulation of normal cell volume and pressure
Inhibition of the pump
- The pump requires binding by Nat, K+ and ATP for its operation. Therefore, if the concentration of any of these substances is too low, the pump does not function.
- When temperature is reduced.
- During lack of oxygen.
- Metabolic poisons which prevent the formation of
АТР.
Calcium (Ca2+) pump or Ca?+ ATPase
(i) The calcium pump is present in the sarcoplasmic reticulum of muscle cells which maintain the intracellular ionic Ca?+ concentration below 0.1 mmol/L.
(ii) It is also located in the cell membrane and in many cell organelle membranes. In the cell membrane, the direction of Ca?+ transport is from cytoplasm to ECF. In cell organelle membranes it is from cytoplasm into the organelle lumen.
This is why the cytoplasm of most cells has a very low Ca?+ concentration.
Potassium-hydrogen (K+ – H*) pump or K+ _ H+ ATPase (or H+ – K+ ATPase)
The potassium-hydrogen pump is present in the cells of the gastric mucosa and renal tubules where it causes the secretion of H+
B. Secondary active transport processes
In some tissues, the active transport of Nat is coupled (linked) with the transport of other substances, i.e. the transport of many ions and nutrients along their electrochemical energy gradients is accomplished by Nat-dependent secondary active transport .
For example: Glucose and amino acids are reabsorbed from the proximal renal tubules or absorbed from the intestinal lumen only if Na+ binds to the protein and is transported down its electrochemical gradient at the same time.
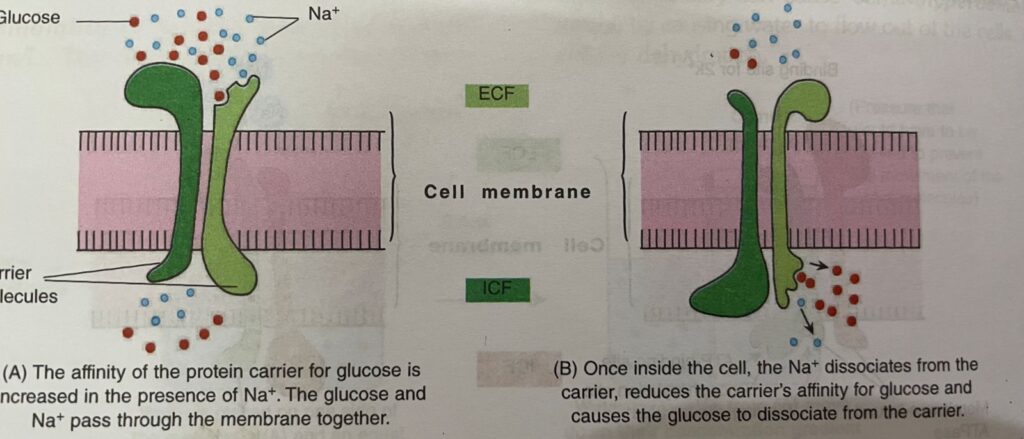
C. Carrier type processes
Carriers are transport proteins. They bind ions and other molecules and then change their shape, moving the bound molecules from one side of the cell membrane to the other.
Types
- Uniporters are carriers which transport a single particle in one direction, such as the facilitated diffusion of glucose.
- Symporters (co-transporters) transport two particles together in the same direction, such as the secondary active transport of glucose (see above).
- Antiporters (counter transporters) transport molecules in opposite directions, i.e. they exchange one substance for another. For example:
(i) Na+ – K+ pump which moves 3Na+ out of the cell in exchange for 2K+ that moves into the cell
(ii) Nat – Ca?+ exchangers in the muscle cells (iii) Na+ – H+ exchangers in the renal tubules

Important Note
Depending on the location, the carrier type processes can be active or passive
D. Vesicular Transport Processes or Transcytosis
Many substances are transported across the cell membrane by endocytosis and exocytosis.
Important Note
If the substance ingested is in solution form, the process is called pinocytosis (cell-drinking).
- 1. Endocytosis: The endocytosis is of two types.
They are:
(i) Phagocytosis (cell eating): Phagocytosis is the process by which extracellular substances bacteria, dead tissue, foreign particles, etc.) are engulfed by the cells.- (ii) Receptor-mediated endocytosis: The material to be transported first binds to a receptor, and then the receptor-substance complex is ingested by endocytosis. For example, transport of iron and cholesterol into the cells.
- 2.Exocytosis: In exocytosis, substances secreted by the cell are trapped within vesicles or granules which fuse with the cell membrane and release their contents to the ECF. This leaves the contents of the vesicles or granules outside the cell and the cell membrane intact. It requires Ca?+ and energy (Fig. 2.8). Hormones, digestive enzymes and synaptic transmitters are examples of substances transported out of the cell by this process.

Important Note
Vesicular transport processes of any kind require metabolic energy in the form of ATP.
Intercellular Communication: Chemical Messengers
Cells communicate with each other via chemical messengers that include amines, amino acids, steroids, polypeptides, lipids, nucleotides, etc. Some messengers move from cell to cell via gap junction without entering the ECF and some messengers are secreted into the ECF to affect the functions of neighbouring cells. The intercellular communication mediated by messengers in the ECF are of three types. They are:
- Neural communication, i.e. communication via synaptic junction.
- Paracrine communication: In paracrine communication products of cells diffuse in the ECF to affect the neighboring cells. In addition, if product of cells bind to receptors on the same cell, it is called autocrine communication.
- Endocrine communication: In endocrine communication hormones reach cells via the circulating blood.
Mode of action of chemical messengers
The mechanisms by which chemical messengers in the ECF bring about changes in cell function are as follows:
(A) Opening or closing ion channels in the cell membrane: Some ligands bind directly to ion channels in the cell membrane and then alter the permeability of membrane to the ion. For example, action of A-ch on motor end plate.
(B) Stimulation of transcription: The chemical messenger acts via cytoplasmic or nuclear receptors to increase transcription of mRNA. Thyroid and steroid hormones exert their effects in this fashion.
(C) Activating phospholipase C: Binding of ligand to its receptor activates phospholipase C on the inner surface of the membrane. Phospholipase C catalyzes the hydrolysis of phosphotidylinositol diphosphate (PIP 2) to form inositol triphosphate (IP) and diacylglycerol (DAG) both of which act as second messengers.
(i) The IP diffuses to the endoplasmic reticulum (ER) where it triggers the release of Ca?+ into the cell to produce physiological effects.
(ii) DAG stays in the cell membrane where it activates protein kinase C to produce physiological effects
to read about structure and function of cell follow the link